Brian's Seismic
FAQ
Copyright © 2004-2009, B. Vanderkolk,
All Rights Reserved

v0.4a - Feb. 28, 2005
Seismic FAQ
Main Table of Contents
- Before the Earthquake
- During the Earthquake
- After the Earthquake
- Plate Tectonics
- Types of Faults
- Focal Mechanisms (aka Beach Balls)
- Magnitude Scales
- Amount of Energy
- Seismometers
- Types of Seismic Waves
- P-waves
- S-waves
- Love waves
- Rayleigh waves
- Liquifaction
- Significant Historical Earthquakes
- New Madrid, Missouri - December 16, 1811; January 23, 1823;
February 7, 1812
- Haicheng, China - Fubruary 4, 1975
- Tangshan, China - July 27, 1976
- Loma Prieta, California - October 18, 1989
- Landers, California - June 28, 1992
- Northridge, California - January 17, 1994
- Kobe, Japan - January 16, 1995
- Izmit, Turkey - August 17, 1999
- Hector Mine, California - October16, 1999
- Parkfield, California - September 28, 2004
- Sumatra, Indonesia - December 26, 2004
- Earthquake dangers of the near future
In this section are some basic
answers to the most common questions.
What
is an earthquake?
The common definition of an earthquake is the shaking of the
ground caused by slippage or rupture of a fault within the Earth's
crust. The word is also used to refer to the breaking of the fault
itself. In this sense, an earthquake ruptures a fault and this results
in seismic waves radiating from the earthquake. It is these seismic
waves that one feels in the form of ground motion.
See also,
What are seismic waves?,
What is a fault?, and
What
causes an earthquake? for more
information.
What
do I do during an earthquake?
Diclaimer: the following information is advice
only. It is not guaranteed to always work. It is also meant to apply to
modern industrialized nations that have and enforce stringent
earthquake codes, such as Japan and the United States. In some
situations and countries, adhering to the following advice verbatem may
actually be the wrong thing to do. You should always examine your
specific situation and decide what actions to take in the event of a
quake BEFORE it happens.
This is just a simple summary. For more information and variations from
this summary, see
During the Earthquake
under
The Details.
- If you are indoors
- Get under a sturdy desk or table and hold on.
- Move away from outside walls, windows, mirrors, fireplaces,
kitchen appliances, hanging fixtures, shelving.
- Do not use elevators.
- If in a crowd, do not panic and rush for the exit.
- If you are outdoors
- Get out into the open.
- Move away from buildings, poles, power lines, or anything else
that may fall over.
- If you are driving in your car
- Gently pull over and stop the car.
- Avoid stopping on or under bridges and overpasses.
- Stay clear of poles, trees, power lines, and if possible,
buildings.
- If in the mountains be aware of possible rockfalls and landslides.
- If near the ocean beware of tsunami.
- Do not wait for a tsunami warning.
- Start heading inland and/or towards higher ground immediately.
- Wait until a tsunami all clear has been issued before return.
- If you see the ocean suddenly recede or rise more than normal,
RUN!
What
do I do after an earthquake?
First of all, try not to panic.
You must be able to think
clearly in the immediate aftermath in order to protect yourself and
others. It's OK to be scared, but going into hysterics will only do
more harm. If someone else panics, do your best to comfort them and
lead them to safety. A panicked person will often times make irrational
decisions that can harm themselves or others. Do not leave them alone
until they have completely calmed down.
If you smell gas, turn off the gas valve.
Inspect for and evacuate heavily damaged buildings.
Do not use the elevator.
Do not make a mad dash for the exits causing a stampede.
Beware of aftershocks. The larger the quake, the larger the aftershocks.
More information can be found in
After The
Earthquake is
Section II - The Details.
How do
I prepare for an earthquake?
If you do nothing else, at
least know what to
do when one strikes. See
What do
I do during an earthquake? for information.
You should have on hand all the
supplies you would need to survive for
a minimum or three days camping, ideally for two weeks or more. You
must realize that after a major earthquake damage to the basic
infrastructure that we all take for granted will be destroyed
or damaged. That means the possibility of no running water, no
electricity, no natural gas, and no sewage. Due to the damage to roads
and other transportation infrastructure, it may take several days for
assistance to arrive in your area.
At a very minimum you should have three days supply of the following
items:
- 2 gallons of water per person per day. (If you have three people,
that's a total of 18 gallons)
- 3 meals per person per day. (For three people, that's 27 meals)
- All necessary prescription medication.
- Food for all your pets.
- Working flashlight.
- Working battery powered radio.
- A basic first aid kit.
You should also know how to turn off
the natural gas at the meter (keep a wrench at the meter), how to turn
off the electricity mains, and how to turn off the water.
For a more detailed discussion of
earthquake preparedness, please see
Before the
Earthquake
in
Section II - The Details.
What are 'seismic waves'?
Seismic waves are what you feel the ground doing in an earthquake. When
the fault ruptures, it sends vibrations out from the fault. These
vibrations are somewhat like the waves radiating from a rock thrown
into a pond. There are many types of seismic waves, most notably
p-waves and s-waves. P-waves are pressure waves moving through the
earth, compressing and expanding the ground as they pass by. S-waves
move the ground form side to side. Also, there are surface waves know
as Love waves and Rayleigh waves.
See
Types
of Seismic Waves in
Section II for more
information.
What is liquifaction?
Liquifaction is when the seemingly solid ground acts more like a
liquid. This is most prevalant when the soil is wet or loosely packed.
When the ground
starts shaking, the vibrations cause the wet soil to lose cohesion and
act more like a fluid. Structures on wet soil may sink or the liquid in
the soil may be forced out of the ground as sand blows.
See
Liquifaction in
Section
II for details.
What
causes an earthquake?
Although we know what an earthquake is and some of the forces
that spawn them, exactly how they start is still an intensive area of
study. We do know that through plate tectonics, large sections of crust
are moving around the surface of the Earth. Where these sections meet
they grind past and even over or under each other. These meeting points
bend and fracture the normally solid earth due to the enormous stresses
resulting in the creation of faults. When the pressure builds enough to
overcome the friction on the fault, the two sides of the fault move
past each other suddenly in the form of an earthquake.
What
is a fault?
A fault is a fructure in the earths crust between two blocks of
crust that are moving relative to each other. There are many types of
faults, each for different types of fault movement.
See
Types of Faults in
Section
II - The Details for specific
information.
What
is plate tectonics?
Plate tectonics is a theory that describes thin plates forming a
solid crust over the more fluid mantle of the Earth. These plates are
in constant motion. Where plates are moving apart, spreading ridges are
formed. Where they slide past each other, fault lines develop. Where
they collide more directly, one slides under the other in a subduction
zone. Plate tectonics also helps to explain the presence of chains of
volcanoes in certain parts of the Earth.
See
Plate Tectonics in
Section
II - The Details for more information.
What
is the Richter Scale?
The Richter Scale was developed by Charles Richter in 1935 at
the California Institute of Technolgy. It was the first reliable way of
comparing the size of earthquakes. The method used a specific type of
seismometer common for the time and was done by measuring the physical
size of the seismogram traces. Then using other information, such as
the distance from the quake, the information was put into a simple
formula and a magnitude number was derived. This scale is logarithmic,
meaning that a one point increase in magnitude corresponded to
approximately a 32 times increase in energy.
The Richter Scale is no longer in use, mainly due to the fact that it
was calibrated to a specific type of seismograph (which is no longer
used) and was also calibrated to the crustal conditions of southern
California, making it less accurate for other parts of the globe. Many
seismologists today cringe when the media continues to say that a
particular earthquake was a given size "on the Richter Scale."
See
Magnitude Scales in
Section
II - The Details for more information on
the many types of magnitude scales in use and their differences.
What
is the Modified Mercalli Intensity
Scale?
The Modified Mercalli Intensity Scale (symbolized as 'MM') is a method
of rating the intensity of an earthquake. The scale has 12 levels
represented by Roman numerals ranging from I to XII and was developed
by Harry Wood and Frank Neumann in 1931. The scale is not
mathematically defined or measurable by instruments. Rather, it is a
subjective measure based on the observed effects of the quake, such as
the extent and severity of damage. It is entirely possible for an
earthquake of a given magnitude to be rated a different Mercalli rating
due to it's location. In a country such as Japan or the United States,
earthquakes do not cause as much damage as the same magnitude
earthquake occuring in a place such as Guatemala or Indonesia. This is
due primarily to better constuction methods and government mandated
building codes. Below is a description of each intensity rating taken
from a USGS page describing the Mercalli scale.
From
http://neic.usgs.gov/neis/general/mercalli.html
- I
- Not felt except by a
very few under
especially favorable conditions.
- II
- Felt only by a few
persons at rest,
especially on upper floors of buildings.
- III
- Felt quite
noticeably by persons indoors,
especially on upper floors of buildings.
Many people do not recognize it as an
earthquake. Standing motor cars may rock slightly. Vibrations similar
to the passing of a truck. Duration estimated.
- IV
- Felt indoors by
many, outdoors by few
during the day. At night, some awakened.
Dishes, windows, doors disturbed; walls
make cracking sound. Sensation like
heavy truck striking building. Standing
motor cars rocked noticeably.
- V
- Felt by nearly
everyone; many awakened.
Some dishes, windows broken. Unstable
objects overturned. Pendulum clocks
may stop.
- VI
- Felt by all, many
frightened. Some heavy
furniture moved; a few instances of fallen plaster. Damage slight.
- VII
- Damage negligible
in buildings of good
design and construction; slight to moderate
in well-built ordinary structures;
considerable damage in poorly built or
badly designed structures; some
chimneys broken.
- VIII
- Damage slight in
specially designed
structures; considerable damage in
ordinary substantial buildings with
partial collapse. Damage great in poorly built
structures. Fall of chimneys, factory
stacks, columns, monuments, walls.
Heavy furniture overturned.
- IX
- Damage considerable
in specially designed
structures; well-designed frame
structures thrown out of plumb. Damage
great in substantial buildings, with partial
collapse. Buildings shifted off foundations.
- X
- Some well-built
wooden structures
destroyed; most masonry and frame
structures destroyed with foundations.
Rails bent.
- XI
- Few, if any
(masonry) structures remain
standing. Bridges destroyed. Rails bent
greatly.
- XII
- Damage total. Lines
of sight and level are
distorted. Objects thrown into the air.
What
is 'moment magnitude'?
Moment magnitude is a specific way of measuring an earthquake
based on how much of the fault slipped and how much it slipped. This is
the magnitude of choice for seismologists and is the most accurate for
the largest earthquakes. However, it is also one of the most difficult
to compute.
See
Magnitude Scales in
Section
II - The Details for more information.
What is an 'epicenter'?
The epicenter of an earthquake
is the point on the surface of
the Earth above the hypocenter. See
What
is a
'hypocenter'? for more information.
What is a 'hypocenter'?
The hypocenter is the point
within the earth where the
earthquake started, or nucleated. Faults are two dimensional structures
within the crust of the Earth. The point at which the fault first
begins to rupture is the hypocenter. Rupturing will continue to radiate
along the fault plane outward from the hypocenter. The epicenter is the
point on the surface of the earth directly above the underground
hypocenter. The hypocenter does not need to be in the center of the
fault rupture. In the case of the the 2004 Parkfield quake, the
hypocenter was at the south end of the section of fault the ruptured.
Rupture started at the hypocenter and propogated almost entirely to the
north, with very little propogation to the south. For large earthquakes
where the fault ruptures for hundreds or even a thousand or more
kilomenters and the hypocenter/epicenter is at one end of the rupture,
the hypocenter/epicenter ploted on a map may be far from the location
of greatest shaking and damage.
How
are earthquakes measured?
Earthquakes are measured in a variety of ways, most basically by
instruments or by their effects.
Seismometers
measure the ground motion caused by an earthquake and help to determine
its magnitude. Also, earthquakes can be measured using the
Mercalli Scale, which ranks earthquakes
on their effects, such as how they were felt and the damage they caused.
What
is a seismometer?
A seismometer, also known as a seismograph, is a device
for recording ground motion during an earthquake. Specifically, they
record ground acceleration, or how quickly the ground is changing its
rate of motion. Seismometer come in many varieties depending on their
purose. Some are designed to only detect small earthquakes locally.
Others to detect larger quakes from a distance. More recently, a type
of seimometer called a strong motion sensor has been developed to
measure the extreme ground motions near the center of a large
earthquake. This is useful for making a quick automated assessment of
where the most damage may have occured and where rescue efforts should
begin to be concentrated.
For information on specific types of seismometers see
Seismometers in
Section
II - The Details.
What
is a 'moment tensor solution' aka 'beach ball'?
Also known as
fault plane
solution and
focal mechanism
solution. This is a way of showing the orientation and motion of
movement on a fault that has experienced an earthquake.
See
Focal Mechanisms in
Section
II - The Details for more information.
How
much energy is there in an earthquake?
To get a good idea of how much energy is released in an
earthquake, take a look at the following chart:
From http://www.seismo.unr.edu/ftp/pub/louie/class/100/magnitude.html
Richter TNT for Seismic Example
Magnitude Energy Yield (approximate)
-1.5 6 ounces Breaking a rock on a lab table
1.0 30 pounds Large Blast at a Construction Site
1.5 320 pounds
2.0 1 ton Large Quarry or Mine Blast
2.5 4.6 tons
3.0 29 tons
3.5 73 tons
4.0 1,000 tons Small Nuclear Weapon
4.5 5,100 tons Average Tornado (total energy)
5.0 32,000 tons
5.5 80,000 tons Little Skull Mtn., NV Quake, 1992
6.0 1 million tons Double Spring Flat, NV Quake, 1994
6.5 5 million tons Northridge, CA Quake, 1994
7.0 32 million tons Hyogo-Ken Nanbu, Japan Quake, 1995; Largest Thermonuclear Weapon
7.5 160 million tons Landers, CA Quake, 1992
8.0 1 billion tons San Francisco, CA Quake, 1906
8.5 5 billion tons Anchorage, AK Quake, 1964
9.0 32 billion tons Chilean Quake, 1960
10.0 1 trillion tons (San-Andreas type fault circling Earth)
12.0 160 trillion tons (Fault Earth in half through center,
OR Earth's daily receipt of solar energy)
For a detailed discussion on how much energy is in an earthquake, with
formulae and tables, see
Amount of Energy
in
Section II - The Details.
What
is the biggest possible earthquake?
The size of an earthquake is directly related to the area of the
fault plane and how much it moves. The bigger the area and the more it
moves, the bigger the earthquake. The largest quakes occur on
subduction zones. To imagine the fault plane of a subduction zone,
imagine a piece of paper laying on its side. The paper has a large
surface area. Faults such as the San Andreas are more like narrow
ribbons laying on edge and therefore have a more limited surface area.
Although a 'mega-quake' of magnitude 10 is theoretically possible, the
probability of such a large quake is extremely small. Since we can
calculate how big a fault plane would be required for such a large
quake, we can compare this to the known faults of the world. The fact
is, there just isn't a fault big enough to make such a quake. It would
take 32 of the recent Sumatra 9.0 earthquakes to equal a magnitude 10.
The largest earthquake on record was a whopping magnitude 9.5 which
occured in Chile on May 22, 1960.
Can a
bunch of smaller earthquakes release
the stress of a big one?
In a word, no. Due to the enormous range of energies involved, it could
take upwards of millions of smaller non-destructive quakes to relieve
the stress of one large one. At the very least, it would take
thousands. Please see
How much energy is
there in an earthquake? for more detail.
Can
earthquakes be predicted?
Although there are many
individuals and organizations who will
claim otherwise, as yet there is no reliable, repeatable, testable
method for predicting earthquakes. Note that
prediction and
forecasting are two different
things. Forecasting is what the reputable scientists do. They state the
probability of an earthquake happening within a region in a given time
frame. A prediction, on the other hand, says that a quake WILL happen
within a given region in a given time frame. The difference is more
than probability versus certainty. The time frame stated in a forecast
is on the order of years or tens of years. A prediction is on the order
of days or months at most.
For more information on why 99% of all prediction methods are scoffed
at by scientists in the seismic community, please read the section on
Science and the Scientific Method.
For more detailed information on quake prediction and various ideas
that have been proposed, see the section on
Predicting
Earthquakes.
- Before the
Earthquake
- During the
Earthquake
- After the Earthquake
- Plate Tectonics
- Types of Faults
- Magnitude Scales
- Amount of Energy
- Seismometers
- Types of Seismic
Waves
- P-waves
- S-waves
- Love waves
- Rayleigh waves
- Liquifaction
- Significant
Historical Earthquakes
- New Madrid, Missouri - December 16, 1811; January 23, 1823;
February 7, 1812
- Haicheng, China - Fubruary 4, 1975
- Tangshan, China - July 27, 1976
- Loma Prieta, California - October 18, 1989
- Landers, California - June 28, 1992
- Northridge, California - January 17, 1994
- Kobe, Japan - January 16, 1995
- Izmit, Turkey - August 17, 1999
- Hector Mine, California - October16, 1999
- Parkfield, California - September 28, 2004
- Sumatra, Indonesia - December 26, 2004
- Earthquake dangers
of the near future
During the Earthquake
Diclaimer: the following information is advice
only. It is not guaranteed to always work. It is also meant to apply to
modern industrialized nations that have and enforce stringent
earthquake codes, such as Japan and the United States. In some
situations and countries, adhering to the following advice verbatem may
actually be the wrong thing to do. You should always examine your
specific situation and decide what actions to take in the event of a
quake BEFORE it happens.
Knowing what to do during an earthquake depends on where you are when
it
strikes. It is also a question best answered BEFORE an earthquake
rather than during. You should take the time to consider your
surroundings and the places you frequent most, the bedroom, family area
(or wherever you spend most of your time at home when not asleep), the
roads you take to work, your work area, and even those stores you visit
regularly. Preparedness can be as much or even more knowing what to do
than it is having emergency supplies. What good is it to have two weeks
of rations if you get killed or injured because you stopped to watch
the view out that beautiful bay window? There is a lot of information
below, but sometimes it's just enough to ask yourself 'what would I do
where I am if there is an earthquake right now?' Ask yourself that
enough and you won't have to think about it when the next big
earthquake strikes. You will already know what to do.
In developed nations that have excellent building codes and
enforcement, the greatest danger during a large earthquake is not from
buildings falling down on you, but rather from all the items within the
building being flung off the walls and shelves and out of the cupboards
and striking you. The house will survive but that beautiful cherry wood
china cabinet is going to fall over if it's not secured to the wall,
then even if it is, the antique dinner ware may still fly off the
shelves if the doors fling open easily.
Indoors
Running outside during a quake you run the
risk of being hit from debris falling off the building, such as
facades, signs, and broken glass. By the time you realize it's a big
earthquake, it's too late to
try to escape the building as the debris is probably already falling.
Move away from glass windows and mirrors. The strong shaking during
more powerful earthquakes causes the glass to bend until it finally
breaks. Unfortunately, the glass doesn't always fall straight down, but
can often blow outward with considerable force. If you've ever bent a
plastic CDROM until it snaps, you've likely found pieces that flew
several feet away from the disc. Glass can do the
same thing.
It used to be said that you should stand in a doorway since this part
of a house or
building is stronger. Although it may be stronger, the problem with
this is the door. During a large quake it
can swing wildly and uncontrollably possibly causing injury from it
striking you. If you are holding onto the door frame you may even get
your fingers pinched off when the door suddenly slams shut. If you do
decide to stand in a doorway, be sure to face the door and make sure it
doesn't swing and hit you, perhaps by holding onto it.
Move away from the outer walls of a building. These are the most likely
to collapse. If you are inside a tall structure, like a skyscraper, the
violent swaying motions of the building during the
quake may throw you out a window. Move towards the core of the building
and take cover as appropriate.
If you are in a crowded area, such as a concert or busy mall, do not
contribute to the innevitable stampede of people rushing for the exits.
Take the nearest cover and stay there.
Avoid elevators and stairwells. If you
are in a stairwell, leave it at the nearest exit and take appropriate
cover. If you are in an elevator, press the emergency stop button. This
will stop the elevator where it is. The chances of the elevator falling
are very close to zero due to the safeguards built in. Once the shaking
stops, try to pry open the doors and exit the elevator. If the doors
are jammed, try the emergency phone. If power has failed it may not be
working. Also, all elevators have roof hatches. It may be possible to
climb on top of the elevator and open the doors to the floor above.
Failing this, try banging on the door and occasionally
hollering for outside help. Above all, remain calm. Help will arrive if
you are stuck. One of the priorities of emergency crews checking a
building is to check the elevators.
There is some debate over getting under a desk. On the one hand,
getting under the desk will help protect you from debris flying around
the room. Computer monitors can slide off a desk and the monitor glass
shatter upon hitting the floor. You are also more protected from
falling overhead lighting, flying glass from windows and mirrors, and
shelving and their contents flying around the room.
However, during the
rescue efforts after the 1985 magnitude 8.0 quake that affected Mexico
City rescue workers were able to crawl through the pancaked buildings
along passages created by what was left of the crushed desks supporting
the debris above. People that were under their desks were injured or
killed when the ceilings above came down crushing the desks on top of
them. It is difficult to say for certainty when one should be under or
next to the desk.
If you are in bed, wrap yourself in the blankets and roll off onto the
floor beside the bed. DO NOT GET UNDER THE BED. If the building should
collapse, the bed will collapse but may help support the debris enough
to create a void space around where you lie. Roll to the side that is
furthest away from any glass that may shatter or items falling off
walls and shelves. Unsecured shelves tend to fall over in quakes so it
is a good idea to avoid them.
Outdoors
If you are outside, move out into the open. Move away from buildings,
especially those with brick and glass facades. Stay away from power
lines and tall trees. If you are in a shopping center the best place to
go is out into the parking lot UNLESS it is a parking structure which
may collapse.
If you are in a downtown area near skyscrapers or other
very tall buildings where there is no open space to move towards, move
to the entranceway of the nearest building but do not enter. Be careful
of choosing an entranceway with a lot of glass. It may be better to
simply crouch or lay down beside a parked vehicle if you cannot get
elsewhere quickly enough.
Driving
If you are in your
car, slowly come to a safe stop on the side
of the
road so as to not cause an accident or lose control of the vehicle.
Avoid stopping on or under bridges or near poles and large trees. These
can fall on top of the car injuring you. STAY IN YOUR CAR.
However, what if you are stopped in traffic under a bridge? If this is
the case, DO NOT try to drive out
from under the bridge. Put the car in park, turn off the engine, get
out of the car and lay flat on the ground next to the car face down
with your hands covering your head and neck. DO NOT GET UNDER THE CAR.
If the bridge should collapse, the car will be crushed by the weight of
the concrete, but there is a very good chance that a void space will be
created next to the car that you will be relatively safe in. If you
cannot get out of your car quickly, lay down across the seats or floor
of the car. You may have to undo your seatbelt to do this. In the 1989
Loma Prieta quake some of the survivors of the Cypress Freeway collapse
were found laying sideways in
their cars when the freeway collapsed on top of them.
Mountains and Ocean
The obvious danger
when in the mountians or hilly areas is the
danger of rock falls and landslides. If you are out hiking, keep your
eye towards uphill for falling debris. It may even be a good idea to
seek a large tree to hide on the downhill side of. Once the quake is
over, immediately hike back out of the area to the trailhead or seek a
ranger station.
The danger of large earthquakes near the ocean is the possibility of a
tsunami. The best bet is to assume a tsunami is on it's way and to move
quickly inland or to higher ground. It is never known immediately after
any quake whether a tsunami has been generated. Often times it may take
several hours to determine this from seismograph information alone. In
areas where there are tsunami detection buoys it may still take tens of
minutes before a warning is sounded. If the source of the tsunami is
close to shore, it may take only a couple minutes before the tsunami
strikes, long before any official warning.
An ominous sign that a tsunami is imminent is abnormally high swells
crashing on shore or the sea receding further out than any low tide.
Sometimes the sea will pull back so far that it exposes a hundred
meters of ocean bottom. Do not be tempted to run out and collect fish
or seashells. RUN AWAY!!!
Another dangerous fact about tsunami is that they are rarely just one
wave. Tsunami are like the ripples from a stone thrown in a pond; there
are multiple waves. Often times the first few waves
are not the worse. The first couple waves crash ashore and people think
it's all over not realizing that in a few minutes even bigger and
deadlier waves will arrive.
Stay inland or on higher ground until it has been officially declared
that there is no tsunami or the tsunami is over and it is safe to
return. Only then should you return to the shoreline.
See
Section IV - Tsunami for more
details.
After the Earthquake
Plate Tectonics
Types
of Faults
The types of faults are categorized by the orientation of the
fault plane relative to level ground and the direction of slip. There
are two basic types of fault, the strike slip and the dip slip. A
strike slip fault is one where the fault plane is vertical and the
predominant motion is side to side. A dip slip fault is one where the
fault plane lies at an angle and the motion is predominantly up and
down. From these two type are various descriptions depending on the
relative motion of each side of the fault.
Dip slip faults have a hanging wall and foot wall. If you view the
fault along its length, the fault dips at an angle. The crustal block
that hangs over the other is the hanging wall and the other is the foot
wall.
Not all faults are purely of one type. Typically they all exhibit
multiple types of movement. The description applied to any particular
fault rupture is usually based on the predominant motion.
I have made several animations to help you visualize the motion and the
focal mechanism (beach ball) associated with that type of fault.
Left Lateral - This is
a strike slip fault where the opposite side moves to the left. That is,
if you are standing facing the fault line, the other side moves to the
left.
Right Lateral - This
is a strike slip fault where the opposite side moves to the right.
That is, if you are standing facing the fault line, the other side
moves to the right.
Normal - This is dip
slip fault where the hanging wall slides down the foot wall.
Reverse - This is a
dip slip fault where the hanging wall slides up the foot wall.
Thrust - This is a
reverse fault where the angle of thrust is less than 45 degrees.
Oblique - An oblique
fault is one that has nearly equal characteristics of both strike slip
and dip slip. The relative motion between each block has both
side-to-side and up-and-down motion.
Subduction - A
subduction fault is a dip slip fault at an extreme angle. They are
almost flat.
Transform - A
transform fault is a strike slip fault and are different only in their
speciic location relative to other faults. They often appear from
spreading ridges and are caused by different parts of the spreading
ridge not spreading at the same rate. Other transform faults lie
between the ends of spreading ridges that are seperated by some
distance.
Here are some sample images of the focal mechanisms for a few types of
fault. In all these images the fault is plane is horizantal (E-W) in
the image. Animations are also available which demonstrate the ground
movement and its associated beach ball symbol.
Focal Mechanisms (aka Beach Balls)
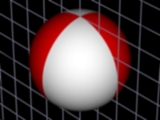
A
focal mechanism is a fancy way of saying which way the fault moved
during an earthquake and is often derived automatically by computer
based analysis of seismograms. A "beach ball" symbol is used to
visualize this
movement. The beach ball is a sphere cut into quarters and shaded with
alternating colors, usually white and some other color. In our example
we use white and red.
The two "slices" through the beach ball represent
the two possible
orientations of the plane of the fault. The computers look at the
waveforms from as many seismographs as possible and by looking at the
direction the ground first starts to move at each location, they can
compute which way the fault moved. Unfortunately, there's always two
possible solutions, each orthogonal to each other. However, it's pretty
easy to determine which fault plane is the correct one by comparing the
computed solutions with the orientation of known faults. One of the
computed solutions usually lines up very closely to the direction of
the fault which produced the earthquake.
The other feature of a beach ball are the colored
quadrants. These
quadrants represent the stress field around the hypocenter, with the
tension axis (where strain is reduced) represented by the white
quadrants, and the pressure axis (where strain increases) represented
by the red quadrants. A simpler way to look at it is that it represents
the direction of motion, that being from white to red.
Sometimes the beach ball is difficult to understand
due to it's
orientation. Sometimes it's just a flat line drawing. Just remember,
the image you are looking at is supposed to
be a 3 dimensional sphere.
Here's an animation demonstrating the direction of
stress orientation with movement. Remember, the red quadrants
represents increasing stress and the white quadrants represents
decreasing stress.
To see more animations demonstrating various fault types and their
associated beach balls, see
Types of
Faults, above.
http://seismo.um.ac.ir/education/Seismic%20Sources.htm
http://serc.carleton.edu/files/NAGTWorkshops/structure04/Focal_mechanism_primer.pdf
http://www.geo.cornell.edu/geology/classes/isacks/fm.pdf
http://quake.seismo.unr.edu/htdocs/WGB/Recent/explanation/
http://bullard.esc.cam.ac.uk/~keith/Physics_Earth_Planet/Lecture_15/physics_lecture_notes_101-114.pdf
http://equake.geol.vt.edu/pdf/4154-source.pdf
Magnitude Scales
http://eqseis.geosc.psu.edu/~cammon/HTML/Classes/IntroQuakes/Notes/earthquake_size.html
http://en.wikipedia.org/wiki/Moment_magnitude_scale
http://earthquake.usgs.gov/image_glossary/seismic_moment.html
from:
http://earthquake.usgs.gov/eqinthenews/2004/usslav/rupture_area.html -
Earthquakes rupture a patch along a fault's
surface. Generally speaking, the larger the rupture patch, the larger
the magnitude of the earthquake. Initial estimates based on the
aftershock distribution show the magnitude 9.0 Sumatra-Andaman Islands
Earthquake ruptured a patch of fault roughly the size of California,
and modeling of the seismic waves show that most of the slip occurred
in the southern 400 kilometers of the patch. For comparison, a
magnitude 5 earthquake would rupture a patch roughly the size of New
York City's Central Park.
Amount of Energy
The range of energy released by
an earthquake is quite large.
The smallest earthquakes (mag 1) are equivilant to a typical blast at a
rock quary. The largest quakes (mag 9) are the equivilent of millions
of nuclear bombs going off at once. This is why magnitude scales are
logarithmic. It's difficult to grasp just how much energy there is in
1,000 megatons. The logarithmic scaling of earthquake magnitudes makes
it much easier to understand by representing them with a small range of
numbers, rarely exceeding 9. However, most people do not understand
mathematical logarithms. There are two common misconceptions about how
magnitude scales. The first is that a difference of one magnitude is a
doubling of magnitude; that is, a magnitude 7 is twice as big as a
magnitude 6. The other misconception is that it is 10 times; that is, a
magnitude 7 is 10 times as big as a magnitude 6.
The reality is that a magnitude 7 is about 32 times as big as a
magnitude 6 in energy. A magnitude 8 is 1,000 times as powerful as a
magnitude 6 quake (32 * 32)!!!
This misunderstanding of the logarithmic nature of earthquake
magnitudes is what leads to the another misconception; that several
smaller quakes can release the energy of a big one, thereby preventing
the big one from occuring. Since the scale is logarithmic, that means
it would take 32 magnitude 6 quakes to relieve the stress of a
potential magnitude 7 quake. I don't knwo about you, but I think I'd
rather experience ONE magnitude 7 quake than 32 magnitude 6's!!! To
continue, it would take 1,000 magnitude 5 quakes, or 32,000 magnitude 4
quakes, or 1 million magnitude 3 quakes to relieve the stress of a
magnitude 7.
If you're wanting to relieve the stress from a potentially devestating
magnitude 9 earthquake, it would take 1 million (1,000,000) magnitude 5
earthquakes to equal the amount of energy in a magnitude 9 quake. Just
one magnitude 5 quake can still cause a lot of damage in lesser
developed areas and be a considerable inconvenience in even the most
prepared and structurally sound cities, but to have to experience a
million of them?
http://eqseis.geosc.psu.edu/~cammon/HTML/Classes/IntroQuakes/Notes/earthquake_size.html
description of moment magnitude & rupture size and offset
From http://www.seismo.unr.edu/ftp/pub/louie/class/100/magnitude.html
Richter TNT for Seismic Example
Magnitude Energy Yield (approximate)
-1.5 6 ounces Breaking a rock on a lab table
1.0 30 pounds Large Blast at a Construction Site
1.5 320 pounds
2.0 1 ton Large Quarry or Mine Blast
2.5 4.6 tons
3.0 29 tons
3.5 73 tons
4.0 1,000 tons Small Nuclear Weapon
4.5 5,100 tons Average Tornado (total energy)
5.0 32,000 tons
5.5 80,000 tons Little Skull Mtn., NV Quake, 1992
6.0 1 million tons Double Spring Flat, NV Quake, 1994
6.5 5 million tons Northridge, CA Quake, 1994
7.0 32 million tons Hyogo-Ken Nanbu, Japan Quake, 1995; Largest Thermonuclear Weapon
7.5 160 million tons Landers, CA Quake, 1992
8.0 1 billion tons San Francisco, CA Quake, 1906
8.5 5 billion tons Anchorage, AK Quake, 1964
9.0 32 billion tons Chilean Quake, 1960
10.0 1 trillion tons (San-Andreas type fault circling Earth)
12.0 160 trillion tons (Fault Earth in half through center,
OR Earth's daily receipt of solar energy)
To put the amount of energy into several different perspectives, a 9.0
magnitude earthquake is equivilant to:
A 32 thousand megaton bomb.
2.13 million Hiroshima bombs.
561 times the largest nuke detonated of 57 megatons.
Equivilant mass/energy conversion (E=mc2) of 24.9 kilograms. (The
largest nuke ever detonated at 57 megatons was 2.7kg)
1000 gigawatts of electricity for the next 72 years.
7.1 billion 100 watt lightbulbs for 100 years.
Total electricity production of US in 2002, for over 161 years.
Total eletricity production of the world in 2001, for over 40 years.
Total energy in sunlight the Earth receives in 4.8 hours.
Amount of energy the Sun produces in 5.8 millionths of a second.
Seismometers
Types of Seismic Waves
http://www.geo.mtu.edu/UPSeis/waves.html
P-Waves
S-Waves
Love Waves
Raleigh Waves
Liquifaction
Significant Historical Earthquakes
The earthquakes included in this section are not significant
because of death-tolls, property damage, or the like. They are included
because their occurence had a significant impact on the science of
seismology. These earthquakes exposed the field to new data that lead
to a greater understanding of the processes involved in earthquakes.
They can also be significant because they dramatically pointed out a
danger previously not given enough attention.
New Madrid, Missouri -
December 16, 1811; January 23, 1823;
February 7, 1812
Three large quakes occur in America's heartland. Are they prepared for
more?
Haicheng, China - Fubruary 4, 1975
A successful prediction?
Tangshan, China - July 27,
1976
A failed prediction.
Loma Prieta, California -
October 18, 1989
A wake up call for the San Francisco Bay Area.
Landers, California - June
28, 1992
The San Andreas is not southern Californias only big quake producing
fault.
Northridge, California -
January 17, 1994
Blind thrust faluts in urban areas.
Kobe, Japan - January 16, 1995
A city that was supposed to be well prepared.
Izmit, Turkey - August 17,
1999
Poor construction, failure to enact and enforce building codes.
Hector Mine, California -
October16, 1999
A triggered quake.
Parkfield, California -
September 28, 2004
The Parkfield Experiment. It finally arrived, if a few years too late.
Sumatra, Indonesia - December
26, 2004
The need for tsunami education and warning systems in all the
worlds oceans.
Earthquake dangers of the near future.
Section III - Predicting Earthquakes
This section is obviously devoted to the topic of predicting
earthquakes, prediction methods, what works and what fails, and why.
Can earthquakes be predicted?
Many people will probably answer YES to this question. The
reality is, not yet. If you ask any seismologist they will almost
certainly say that prediction would be wonderful to obtain, but almost
always agree that it is some time off in the future. Of all the people
who claim to be able to predict quakes, very few are willing to
seriously discuss and answer the inevitable questions raised by such a
bold claim. Many are unwilling to discuss their methods. Some of them
are only willing to discuss their methods with large infusions of
'research funds.' Some even make their methods available through the
purchase of their books and equipment. Yes, I am implying they are
probably selling snake-oil, only being interested in making a profit
instead of truly helping humanity protect themselves from the wrath of
Mother Nature. Such people become vehemently defensive when their
motives come into question.
But to get more in line with science, many seismologists feel that
prediction could just be a matter of knowledge. We simply do not yet
know enough of the details of the inner workings of Mother Earth in
order to predict what she will do next. Some will even say we may never
be able to truly predict quakes because the computational requirements
to take into account enough details are beyond any computing power we
can currently envision, but that we may be able to shorten our
forecasts to the same level as current weather forecasts. Other will
say it's entirely impossible due to the forces of chaos acting on a
such indeterminant systems.
Prediction vs. Forecast
A prediction and a forecast have much in common, but they often differ
in their scale, generally the prediction being more precise.
The main difference between a prediction and a forecast is one of
probability. Nearly all predictors say the quake WILL happen, a
probability of 100%. They are nearly 100% wrong as well. A forecast on
the other hand simply states the percent likelyhood of a quake occuring
in the given time span, usually on the order of decades. the forecast
is much more accurate because it is usually based on the seismological
history of the region.
A prediction and a forecast usually do not give the exact location of
the expected eathquake. Seismologists forecast quakes for specific
faults more often than for an entire region. If they do give a regional
forecast, it is based on a summary of forecasts for the specific faults
in that region. Predictions on the other hand tend to be very broad and
imprecise in their location. A seismologist will give a forcast for the
southern segment of the San Andreas whereas a predictor will simply say
'somewhere in Southern California.' The problem is that there are
dozens of major faults in Southern California. This is a case where the
seismologist generally is more precise than the predictor.
The time of the expected quake is expressed very differently between
predictions and forecasts. The predictor tends to give precise dates
and times with a time window on the order of days. For example, they
may say quake will occur within 3 days of January 17, 2000. That means
the quake can occur anywhere from the 14th through the 20th. A forecast
provides a much larger time window, more likely stating that the quake
has a 50% probablity of occuring within the next 15 years.
The methods of deriving predictions and forecasts almost couldn't be
greater. A forecast is based on the seismological history of the region
or fault. If a fault shows a history of rupturing every 50 years on
average, and it's been 40 years since the last rupture, the forecast
will probably say somehting like "a 50% probability of an earthquake
happening within the next 10 years." Predictions, on the other hand,
are derived from as many varied sources as there are predictors. The
most common methods seem to involve 'scalar waves', earthquake clouds,
animal behaviour, astrology, planetary alignments, lunar effects,
visions, and probably a dose of tea leaf reading as well.
Are
scientists looking for ways to predict quakes?
Keilis-Borok
Earthquake Clouds
Some claim that prior to an earthquake, certain types of clouds
form over the region. Typically, these clouds are described as having a
wavelike pattern to them, like ripples in the sand. To use the correct
meterological term, they are undulatus in form. Some say these clouds
are caused by radon gas escaping from the Earth whereas others claim
they are formed from
scalar waves.
Radon - Radon is
element number 86 on the periodic table, one of the noble gases. It is
colorless, oderless, and the heaviest element that can be gaseous at
room temperature. Radon is the decay product of other radioactive
elements, mainly radium and actinium, decaying primarily into polonium.
The half life of radon is very short, the longest lived isotope being
222Rn
with a half life of only 3.8235 days. This is also the most common form
of radon found in nature. Being element number 89 on the periodic table
makes radon a very heavy element, heavier than even gold or lead. This
means radon gas is heavier than air and collects in low lying
unventilated areas such as basements. Being a radioactive gas, radon is
a health hazard and is considered the second leading cause of lung
cancer in the United States. For more information on the health risks
of radon, please visit the
Environmental
Protection Agency website. People are encouraged to obtain radon
detection kits to determine their risk. Free ones are available. The
abundance of radon in the atmosphere is 1 part per 10
21,
or 1 atom of radon for every 1,000,000,000,000,000,000,000 molecules of
air.
Now that we know something more about radon, we can ask a lot of
questions about radon's role in forming earthquake clouds.
- How does radon cause water vapor in the atmosphere to condense
and form clouds?
- Since radon has such a low abundance, how can there be enough of
it to form clouds?
- If large pockets of radon gas suddenly form and are released,
what causes this sudden build up of radon? Remember, radon's half life
is only 3.8 days so it can't accumulate unless there is a concentrated
source of radium that suddenly decides to decay into radon.
- Since radon is heavier than air, how does it manage to get
thousands or even tens of thousands of feet into the air to form
earthquake clouds?
- Why isn't there always a lot of earthquakes in those areas where
radon gas is abundant?
- Why isn't there always a lot of radon in those areas where there
a lot of earthquakes?
- Why hasn't anyone ever detected all this radon, or it's parent
nuclides, or it's decay products?
Further thinking also reveals a couple questions about earthquake
clouds in general, regardless of radon's role.
- Why aren't there always earthquakes when earthquake clouds form?
- Why aren't there always earthquake clouds when there are
earthquakes?
Undulatus Cloud Formation
- Clouds are formed when a mass of air containing water vapor cools,
causing the vapor to condense into small droplets which scatter and
reflect light. This is generally caused by a mass of moist air being
forced to rise higher into the atmosphere. As the altitude increases,
the pressure and temperature drops, increasing the relatice humidity
level until it reaches 100% and condensation forms. Sheets of clouds
can also form where two overlying air masses meet. The lower air mass
may be warm and moist whereas the overlying air mass is cold and dry.
Where these too air masses meet there is some mixing of the air and
clouds form. Undulatus formations occur when these two air masses are
moving at slightly different speeds relative to each other. As the air
masses move across each other turbulence forms and at the right
relative speeds can form organized undulations. These undulations
control the zones where more or less vapor condenses producing the
undulatus formation.
Planetary Alignments
Some argue that the alignments of the planets have a
gravitational effect that can cause or influence earthquakes. Although
every body in the solar system is attracted to every other body by
gravity, gravity is not as powerful as one might like to think. Of the
four fundmental physical forces - gravity, electromagnitism, and the
weak and strong nuclear forces - gravity is by far the weakest of the
bunch. The weak and strong nuclear forces fall off very rapidly and
only have effective ranges within the nucleus of an atom; it's what
holds them together! Electromagnetism and gravity fall off much more
slowly (inverse-square) and can be detected across the entire universe.
Gravity, though, is 10
36 times
weaker than electromagnetism. This means that at short distances,
electromagnetism wins out. Gravity only wins when at least one body is
a large mass or when one mass is very dense and the other is very
close. This is why even a small magnet can defy gravity and pick up
small objects. Although the Earth's mass is large compared to the
magnet, gravity is so weak that at close enough ranges, the magnetic
field can overpower gravity and still pick up that piece of metal.
In the Solar System, the body with the most gravitational influence on
the Earth is of course the Sun. The Moon is next but the force is about
165 times weaker. The maximum possible gravitational attraction between
the Earth and other major bodies in the Solar System are summarized in
the table below, ranked in order of greatest possible attraction.
Rank
|
Body
|
Times Weaker
Than Sun
|
Times Weaker
Than Moon
|
1
|
Sun
|
reference = 1
|
0.006063
|
2
|
Moon
|
165
|
reference = 1
|
3
|
Jupiter
|
16,775
|
102
|
4
|
Venus
|
27,487
|
167
|
5
|
Saturn
|
231,829
|
1,406
|
6
|
Mars
|
426,096
|
2,583
|
7
|
Mercury
|
1,662,588 |
10,080
|
8
|
Uranus
|
7,082,751
|
42,942
|
9
|
Neptune
|
16,595,541,132
|
100,617,515
|
10
|
Pluto
|
112,511,698,279 |
682,149,944
|
As can be seen from the above table, the gravitational influence of the
planets is much weaker than the Moon and many times weaker still than
the Sun. Even if you added up the attraction of all the other planets
the combined result would still be many dozens of times weaker than the
Moon's influence. Studies have been done to look for a correlation
between earthquakes and the Moon and have found at best only a very
weak relationship, and that small amount is still
under debate. Since we know the Moon has little to no influence, how
could the planets have any at all? For information, see the part on
Lunar Influence.
Astrology
Lunar Influence
Ocean Tides
Scalar Waves
Scalar waves is the brainchild of a one Thomas E. Bearden who
first proposed them beginning in the 1980's. These waves are supposed
to be a form of electromagnetic energy that hase been described
variously as an energy transfer on another plane, another dimension,
subspace, or hyperspace. Many people have proposed theories using
scalar waves to explain various phenomenon, including that there are
scalar waves emanating from the Earth's crust prior to an earthquake.
Various 'instruments' have been devised to measure and record this
scalar energy and proper analysis is supposed to be able to predict
impending quakes.
The fact is, after researching it, there is no such thing as scalar
waves. It appears to be the result of a misunderstanding of the not
often used quaternion form of Maxwell's EM equations. I barely
understand quaternions myself, so I won't pretend to explain them here.
The upshot is that it reduces Maxwell's set of 4 vector equations down
to 2. They are mathematically identical, just written differently and
more compactly. Some have failed to realize the two forms are identical
but instead think the quaternion form leads to a different physics than
the normally used vector form. This misunderstanding is the apparent
basis of 'scalar electromagnetics.'
BTW, a scalar is simply a value without dimension. They have magnitude,
but not direction. Examples of a scalar quantity are mass, speed,
temperature, and time. Electromagnetic waves have both scalar and
vector quantities, that is, both magnitude and direction. Literally, a
'scalar wave' would only have magnitude and no direction. The 'wave'
doesn't go anywhere, so how can there be any energy transfer?
For further information, here's some wikipedia articles:
http://en.wikipedia.org/wiki/Maxwell%27s_equations
http://en.wikipedia.org/wiki/Quaternion
http://en.wikipedia.org/wiki/Scalar
This simple conclusion is that any theory based on 'scalar waves' is
based on a fundamental error and is therefore fundamentally flawed. It
would behoove the authors of theories based on scalar waves to rethink
their theories without the use of these imaginary waves.
Earthquakes Triggering Other Earthquakes?
What
is a tsunami?
Tsunami is a Japanese
word meaning "harbor wave." A tsunami should not be confused with
the word
tidal wave. A tidal
wave is by definition a wave caused by ocean tides, which in turn is
caused by the Moon and a little by the Sun. A tsunami is specifically
caused by an earthquake, landslide (both undersea and from land into
water), or even from an asteroid hitting the ocean. Generally, a
tsunami is almost always cause by an earthquake under water.
What causes a tsunami?
A tsunami can be caused by several things; an underwater
landslide, a landslide falling into water, an asteroid impact, an
exploding volcano, but most notably and underwater earthquake.
When an earthquake occurs, the land shifts and permanently changes
position. If the land moves from side to side, not much will happen to
the water. However, if the land moves up or down, it displaces a large
body of water. Since water seeks to level itself, this displaced water
will attempt to adjust itself in the form of a wave. What makes a
tsunami so special is that this wave extends from the surface of the
water to the bottom of the ocean. Your common everyday wave that
crashes on your favorite beach is only moving water in the top few
meters of water. Deeper down the water remains relatively immobile and
unaffected. But a tsunami affects the whole mass of water above the
land that moved. This translates into a large amount of water being
displaced.
The waves from a tsunami radiate outward and can travel a great
distance. This is because the period (the time from crest to crest) is
extremely long. Most waves crashing on a beach have periods of tens of
seconds whereas a tsunami has periods of minutes to hours. Also,
tsunami waves out in the open ocean move at a very high rate of speed,
as much as 500 kilometers/hour. This may seem astonishingly fast, but
the waveheight out in the open ocean is typically only a few tens of
centimeters. If you were in a boat in the middle of the Pacific when a
tsunami went by, you wouldn't even notice it amongst all the other wave
action.
What makes the tsunami dangerous compared to tide and wind driven waves
is what happens when they reach shallower waters on their way to land.
As anyone who's been to the beach has noticed, far from shore the waves
are gentle rolling swells. As a wave approached shallower water, the
wave is forced to slow down, which causes the wave to pile up on
itself. Eventually the wave piles high enough that it crashes over on
itself. The remains of this crashed wave then runs up the slope of the
beach to a level several feet above the current ocean level. A tsunami
is effectively a large swell. As it approaches shallower water, it also
slows down and piles up. However, they don't come crashing ashore like
portrayed in the movies, nor like the big 50 footers surfers love in
Hawaii. Instead, a tsunami wave simply acts as a sudden rising of water
level, like an extreme tide but happening in seconds. In a way it's
like a fast moving flood but from the ocean instead of a river. This
rising water level happens so fast that the water rushes inland very
quickly, knocking down all but the sturdiest of structures and carrying
the debris inland. When the water has run up as far as it can go, it
starts rushing back towards the ocean carrying all the debris with it.
A tsunami is never just one wave. Like a pebble dropped in a pond, a
tsunami is several waves in a row. The first is rarely the biggest.
This can be dangerous because people may see the first wave and think
all is over when a few minutes later the next bigger wave comes
ashore...then the next...and the next....
Landslides, asteroids, and exploding volcanoes can also displace large
volumes of water generating a tsunami.
Do all earthquakes cause tsunami?
Not all earthquakes cause tsunami. Well, most cause at least a
small local tsunami. For a large ocean crossing killer to be generated,
a large land mass under the water must move up or down in order to
displace a large volume of water. This is most common in subduction
zones, where one tectonic plate slides under or over the other. If a
strike-slip quake occurs, the land moves from side to side. This does
not displace much if any water and therefore any tsunami is likely to
be small and local.
What do I do in the event of a tsunami?
If you are caught in a tsunami, there isn't much you can do. The
force of the water is just too great to swim against. It can even be so
swift as to make it impossible to hold on to a pole or tree. In fact,
that pole or tree is likely to be ripped from the ground in a larger
tsunami. The best thing you can do is at the least sign of a tsunami is
to run inland or to higher ground. Get on top of anything to get out of
the water. Don't wait for the water to consume you.
If you are near the ocean and you feel a large quake, you should assume
that a tsunami is coming and move inland to higher ground immediately.
If the ocean suddenly recedes or rises more than normal, even if you
haven't felt a quake, it could be a tsunami and you should react
accordingly. Hopefully the first wave will not be the worst and it will
serve as a warning for you to seek safety.
How do I prepare for a tsunami?
The best preperation for a tsunami is to know the warning signs
and what to do before the waves arrive. In areas that are prone to
tsunami danger, plan your evacuation route in advance. Know where
higher ground is.
If you are near the ocean and you feel a large quake, you should assume
that a tsunami is coming and move inland to higher ground immediately.
If the ocean suddenly recedes or rises more than normal, even if you
haven't felt a quake, it could be a tsunami and you should react
accordingly. Hopefully the first wave will not be the worst and it will
serve as a warning for you to seek safety.
Can tsunami be predicted?
Tsunami can't be predicted per se, but we do know what causes
them. Large earthquakes can be detected and if the ground movement is
such that a tsunami might be generated, a warning can be issued. But
this takes time and many times it can be too long to help. Special
buoys can be placed out in the open ocean that are designed to detect
the large pressure wave of a tsunami as it passes. If these buoys are
placed in known danger zones, they can help detect the tsunami long
before the seismographs tell us that a large quake of the right type to
cause a tsunami has occured. This information can then be relayed to
the proper government agencies and evacuation orders given. But this is
all predicated on a system being in place, proper communication
channels established, evacuation plans put in place, and the public
educated.
Mega-tsunami.
Indian Ocean tsunami from Sumatra 9.0
on Dec. 26, 2004
Why wasn't there any warning?
Why was the tsunami so big?
http://earthquake.usgs.gov/eqinthenews/2004/usslav/neic_slav_faq.html
http://www.noaanews.noaa.gov/stories2004/s2358.htm
Mt. Saint Helens
Mt. Rainier
Hawaii
Las Palmas
Section VI - Science and the Scientific Method
I have included a section of science and the scientific method in order
to help educate the public in the ways that scientists think and the
logic used to examine evidence and produce theories. Nearly every time
a major disaster occurs, people begin questioning and even blaming the
scientists. It is my opinion that this attitude towards science and
scientists is due simply to a lack of education. Science is not
something that always comes naturally. That's why most go to school for
years to learn this stuff. I hope with this section I can provide a
synopsis of the fundamentals of science that can be read and hopefully
understood by nearly everyone without the need for four years of
university.
This section is also useful for those who, to be blunt, think they are
smarter than the scientists and think they have a theory that explains
everything. For these individuals, I suggest jumping straight to
Why People Believe Weird Things, do
not pass go, do not collect $200. Actually, everyone should read this
and humble themselves. I found it to be a real eye opener myself.
What is the scientific method?
The scientific method is a fundamental procedure for conducting
science composed of four basic steps. I have seen this worded in many
different ways, but they all fundamentally mean the same thing. Here it
is in my own words:
- Observation - This is the process of taking measurements of the
subject in question and defining the nature of the problem.
- Hypothesis - Form a theory that explains the observations based
on previously known and accepted ideas.
- Prediction - Using the hypothesis, deduce the possibility of
potential observations not yet known.
- Experiment - Design and perform a test to verify or refute the
predictions.
Generally, this is an
iterative process, that is, it is done over and over and over. The
results of the experiment are combined with the previous observations
and the whole lot goes through the process again.
If the results of the test verify the predictions of the hypothesis,
the theory is supported. The hypothesis remains the same, but new
predictions must be made to attempt to find holes in the theory.
If the results refute the predictions of the hypothesis, the hypothesis
is in error. At this point, the hypothesis is adjusted to explain both
the previous observations and the new observations.
Why
People Believe Weird Things
The following section is quoted from chapter three of Michael Shermer's
book
Why People Believe Weird Things.
The chapter, titled
How Thinking
Goes Wrong, contains a list of "Twenty-five Fallacies That Lead
Us to Believe Weird Things". These 25 Fallacies cover nearly every
aspect of how non-skeptics, non-critical thinkers, pseudoscientists,
and yes, even scientists, fail in their thinking processes and end up
accepting conclusions based on incorrect assertions and false logic.
Also presented are Hume's Maxim and Spinoza's Dictum, both important
tools used in critical thinking.
I am grateful to Michael Shermer for graciously giving me permission to
quote this material from his book. Michael Shermer is the founding
publisher of
Skeptic Magazine
and director of the Skeptics Society (
www.skeptic.com).
In addition to writing several books, he is also a contributing editor
and monthly columnist for the magazine
Scientific American (
www.scientificamerican.com).
My purpose for including this text is to help educate the public in the
ways of critical thinking used by scientists. It is also here as
reference material for the section
Predicting
Earthquakes.
Hume's
Maxim
Skeptics owe a lot to the Scottish
philosopher David Hume (1711-1776), whose An Enquiry Concerning Human Understanding
is a classic in the skeptical analysis. The work was first published
anonymously in London in 1739 as A
Treatise of Human Nature. In Hume's words, it "fell dead-born
from the press, without reaching such distinction as even to excite a
murmur among the zealots." Hume blamed is own writing style and
reworked the manuscript into An
Abstract of a Treatise of Human Nature, published in 1740, and
then into Philosophical Essays
Concerning the Human Understanding, published in 1748. The work
still garnered no recognition, so in 1758 he brought out the final
version, under the title An Enquiry
Concerning Human Understanding, which today we regard as his
greatest philosophical work.
Hume distinguished between
"antecedent skepticism," such as René Descartes' method of
doubting everything that has no "antecedent" infallible criterion for
belief; and "consequent skepticism," the method Hume employed, which
recognizes the "consequences" of our fallible senses but corrects them
through reason: "A wise man proportions his belief to the evidence."
Better words could not be found for a skeptical motto.
Even more important is Hume's
foolproof, when-all-else-fails analysis of miraculous claims. For when
one is confronted by a true believer whose apparently supernatural or
paranormal claim has no immediately apparent natural explanation, Hume
provides an argument that he thought so important that he placed his
own words in quotes and called them a maxim:
The plain consequence is (and it is a general maxim worthy of our
attention), "That no testimony is sufficient to establish a miracle,
unless the testimony be of such a kind, that it's falsehood would be
more miraculous than the fact which it endeavors to establish."
When anyone
tells me that he saw a dead man restored to life, I immediately
consider with myself whether it be more probable, that this person
should either deceive or be deceived, or that the fact, which he
relates, should really have happened. I weigh the one miracle against
the other; and according to the superiority, which I discover, I
pronounce my decision, and always reject the greater miracle. If the
falsehood of his testimony would be more miraculous than the event
which he relates, then, and not till then, can he pretend to command my
belief or opinion. ([1758] 1952, p.491)
Problems in Scientific Thinking
1.
Theory Influences Observations
About the human quest to understand the physical world,
physicist and Nobel Laureate Werner Heisenberg concluded, "What we
observe is not nature itself but nature exposed to out method of
questioning." In quantum mechanics, this notion has been formalized as
the "Copenhagen interpretation" of quantum action: "a probability
function does not prescribe a certain event but describes a continuum
of possible events until a measurement interferes with the isolation of
the system and a single event is actualized" (in Weaver, 1987, p. 412).
The Copenhagen interpretation eliminates the one-to-one correlation
between theory and reality. The theory in part the reality. Reality
exists independent of the observer, of course, but out perceptions of
reality are influenced by the theories framing our examination of it.
Thus, philosophers call science theory
laden.
That theory shapes perceptions of
reality is true not only for quantum physics but also for all
observations of the world. When Columbus arrived in the New World, he
had a theory that he was in Asia and proceeded to perceive the New
World as such. Cinnamon was a vluable Asian spice, and the first New
World shrub that smelled like cinnamon was declared to be it. When he encounter the
aromatic gumbo-limbo tree of the West Indies, Columbus concluded it was
an Asian species similar to the mastic tree of the Mediterranean. A New
World nut was matched with Marco Polo's description of a coconut.
Columbus's surgeon even declared, based on some Caribbean roots his men
uncovered, that he had found Chinese rhubarb. A theory of Asia produced
observations of Asia, even though Columbus was half a world away. Such
is the power of theory.
2.
The Observer Changes the Observed
Physicist John Archibald Wheeler noted, "Even to observe so
minuscule an object as an electron, [a physicist] must shatter the
glass. He must reach in. He must install his chosen measuring
equipment.... Moreover, the measurement changes the state of the
electron. The universe will never afterward be the same" (in Weaver
1987, p. 427). In other words, the act of studying an event can change
it. Social scientists often encounter this phenomenon. Anthropologists
know that when they study a tribe, the behavior of the members may be
altered by the fact they are being observed by an outsider. Subjects in
a psychology experiment may alter their behavior if they know what
experimental hypotheses are being tested. This is why psychologists use
blind and double-blind controls. Lack of such controls is often found
in tests of paranormal powers and is one of the classic ways that
thinking goes wrong in the pseudosciences. Science tries to minimize
and acknowledge the effects of the observation on the behavior of the
observed; pseudoscience does not.
3.
Equipment Constructs Results
The equipment used in an experiment often determines the
results. The size of our telescopes, for example, has shaped and
reshaped our theories about the size of the universe. In the twentieth
century, Edwin Hubble's 60- and 100-inch telescopes on Mt. Wilson in
southern California for the first time provided enough seeing power for
astronomers to distinguish individual stars in other galaxies, thus
proving that those fuzzy objects called nebulas that we thought were in
our galaxy were actually separate galaxies. In the nineteenth century,
craniometry defined intelligence as brain size and instruments were
design that measured it as such; today intelligence is defined by
facility with certain developmental tasks and is measured by another
instrument, the IQ test. Sir Arthur Stanley Eddington illustrated the
problem with this clever analogy:
Let
us suppose that an ichthyologist is exploring the life of the ocean. He
casts a net into the water and brings up a fishy assortment. Surveying
his catch, he proceeds in the usual manner of a scientist to
systematize what it reveals. He arrives at two generalizations:
(1) No sea-creature is less than two inches long.
(2) All sea-creatures have gills.
In applying this analogy, the catch stands for the
body of knowledge which constitutes physical science, and the net for
the sensory and intellectual equipment which we use in obtaining it.
The casting of the net corresponds to observations.
An onlooker may object that the first generalization
is wrong. "There are plenty of sea-creatures under two inches long,
only your net is not adapted to catch them." The ichthyologist
dismisses this objection contemptuously. "Anything uncatchable by my
net is ipso facto outside of
the scope of ichthyological knowledge, and is not part of the kingdom
of fishes which has been defined as the theme of ichthyological
knowledge. In short, what my net can't catch isn't fish." (1958, p.16)
Likewise, what my telescope can't see isn't there, and what my
test can't measure isn't intelligence. Obviously, galaxies and
intelligence exist, but how we measure and understand them is highly
influenced by our equipment.
Problems in Pseudoscientific
Thinking
4.
Anecdotes Do Not Make A Science
Anecdotes - stories recounted in support of a claim - do not make a
science. WIthout corroborative evidence from other sources, or physical
proof of some sort, ten anecdotes are no better than one, and a hundred
anecdotes are no better than ten. Anecdotes are told by fallible human
storytellers. Farmer Bob in Puckerbrush, Kansas, may be an honest,
church-going, family man not obviously subject to delusions, but we
need physical evidence of an alien spacecraft or alien bodies, not just
a story about landings and abductions at 3:00 A.M. on a deserted
country road. Likewise with many medical claims. Stories about how your
Aunt Mary's cancer was cured by watching Marx brothers movies or taking
liver extract from castrated chickens are meaningless. The cancer might
have gone into remission on its own, which some cancers do; or it might
have been misdiagnosed; or, or, or.... What we need are controlled
experiments, not anecdotes. We need 100 subjects with cancer, all
properly diagnosed and matched. Then we need 25 of the subjects to
watch Marx brothers movies, 25 to watch Alfred Hitchcock movies, 25 to
watch the news, and 25 to watch nothing. Then we need to deduct the
average rate or remission for this type of cancer and then analyze the
data for statistically significant differences between the groups. If
there are statistically significant differences, we better get
confirmation from other scientists who have conducted their own
experiments separate from ours before we hold a press conference to
announce the cure for cancer.
5.
Scientific Language Does Not Make a Science
Dressing up a belief system in the trappings of science by using
scientistic language and jargon, as in "creation-science," means
nothing without evidence, experimental testing, and corroboration.
Because science has such a powerful mystique in our society, those who
wish to gain respectability but do not have any evidence try to do an
end run around the missing evidence by looking and sounding
"scientific." Here is a classic example from a New Age Column in the Santa Monica News: "This planet has
been slumbering for eons and with the inception of higher energy
frequencies is about to awaken in terms of consciousness and
spirituality. Masters of limitation and masters of divination use the
same creative force to manifest their realities, however, one moves in
a downward spiral and the latter moves in an upward spiral, each
increasing the resonant vibration inherent in them." How's that again?
I have no idea what this means, but it has the language components of a
physics experiment: "higher energy frequencies," "downward and upward
spirals," and "resonant vibration." Yet these phrases mean nothing
because they have no precise and operational definitions. How do you
measure a planet's higher energy frequencies or the resonant vibration
of masters of divination? For that matter, what is a master of divination?
6.
Bold Statements Do Not Make Claims True
Something is probably pseudoscientific if enormous claims are made for
its power and veracity but supportive evidence is scarce as hen's
teeth. L. Ron Hubbard, for example, opens his Dianetics:
The Modern Science of Mental Health, with this statement:
"The creation of Dianetics is a milestone for man comparable to his
discovery of fire and superior to all his invention of the wheel and
arch" (in Gardner 1952, p.263). Sexual energy guru Wilhelm Reich called
his theory of Orgonomy "a revolution in biology and psychology
comparable to the Copernican Revolution" (in Garnder 1952, p.259). I
have a think file of papers and letters from obscure authors filled
with such outlandish claims (I call it the "Theories of Everything"
file). Scientists sometimes make this mistake, too, as we saw at 1:00
P.M., on March 23, 1989, when Stanley Pons and Martin Fleischmann held
a press conference to announce to the world that they had made cold
nuclear fusion work. Gary Taube's excellent book about the cold fusion
debacle, appropriately named Bad
Science (1993), thoroughly examines the implications of this
incident. Maybe fifty years of physics will be proved wrong by one
experiment, but don't throw out your furnace until that experiment has
been replicated. The moral is that the more extraordinary the claim,
the more extraordinarily well-tested the evidence must be.
7.
Heresy Does Not Equal Correctness
They laughed at Copernicus. They laughed at the Wright brothers. Yes,
well, they laughed at the Marx brothers. Being laughed at does not mean
you are right. Wilhelm Reich compared himself to Peer Gynt, the
unconventional genius out of step with society, and misunderstood and
ridiculed as a heretic until proven right: "Whatever you have done to
me or will do to me in the future, whether you glorify me as a genius
or put me in a mental institution, whether you adore me as your savior
or hang me as a spy, sooner or later necessity will force you to
comprehend that I have discovered the laws of living" (in Gardner 1952,
p.259). Reprinted in the January/February 1996 issue of the Journal of Historical Review, the
organ of Holocaust denial, is a famous quote from the
nineteenth-century German philosopher Arthur Schopenhauer, which is
quoted often by those on the margins: "All truth passes through three
stages. First, it is ridiculed. Second, it is violently opposed. Third,
it is accepted as self-evident." But "all truth" does not pass through
these stages. Lots of true ideas are accepted without ridicule or
opposition, violent or otherwise. Einstein's theory of relativity was
largely ignored until 1919, when experimental evidence proved him
right. He was not ridiculed, and no one violently opposed his ideas.
The Schopenhauer quote is just a rationalization, a fancy way for those
who are ridiculed or violently opposed to say, "See, I must be right".
Not so.
History is replete with tales of the lone scientist
working in spite of his peers and flying in the face of the doctrines
of his or her own field of study. Most of them turned out to be wrong
and we do not remember their names. For every Galileo shown the
instruments of torture for advocating a scientific truth, there are a
thousand (or ten thousand) unknowns whose "truths" never pass muster
with other scientists. The scientific community cannot be expected to
test every fantastic claim that comes along, especially when so many
are logically inconsistent. If you want to do science, you have to
learn to play the game of science. This involves getting to know the
scientists in your field, exchanging data and ideas with colleagues
informally, and formally presenting your results in conference papers,
peer-reviewed journals, books, and the like.
8.
Burden of Proof
Who has to prove what to whom? The person making the extraordinary
claim has the burden of proving to the experts and to the community at
large that his or her belief has more validity than the one almost
everyone else accepts. You have to lobby for your opinion to be heard.
Then you have to marshal experts on your side so you can convince the
majority to support your claim over the one they have always supported.
Finally, when you are in the majority, the burden of proof switches to
the outsider who wants to challenge you with his or her unusual claim.
Evolutionists had the burden of proof for half a century after Darwin,
but now the burden of proof is on creationists. It is up to
creationists to show why the theory of evolution is wrong and why
creationism is right, and it is not up to the evolutionists to defend
evolution. The burden of proof is on the Holocaust deniers to prove the
Holocaust did not happen, not on Holocaust historians to prove that it
did. The rationale for this is that mountains of evidence prove that
both evolution and the Holocaust are facts. In other words, it is not
enough to have the evidence. You must convince others of the validity
of your evidence. And when you are an outsider this is the price you
pay, regardless of whether you are right or wrong.
9.
Rumors Do Not Equal Reality
Rumors begin with "I read somewhere that..." or "I heard from
someone that...." Before long the rumor becomes reality, as "I know
that..." passes from person to person. Rumors may be true, of course, but usually
they are not. They do make for great tales, however. There is the "true
story" of the escaped maniac with a prosthetic hook who haunts the
lover's lanes of America. There is the legend of "The Vanishing
Hitchhiker," in which a driver picks up a hitchhiker who vanishes from
his car along with his jacket; locals then tell the driver that his
hitchhiking woman had died that same day the year before, and
eventually he discovers his jacket on her grave. Such stories spread
fast and never die.
Caltech historian of science Dan Kevles once told a
story he suspected was apocryphal at a dinner party. Two students did
not get back from a ski trip in time to take their final exam because
the activities of the previous day had extended well into the night.
They told their professor that they had gotten a flat tire, so he gave
them a makeup final the next day. Placing the students in separate
rooms, he asked them just two questions: (1) "For 5 points, what is the
chemical formula for water?" (2) "For 95 points, which tire?" Two of
the dinner guests had heard a vaguely similar story. The next day I
repeated the story to my students and before I got to the punch line,
three of them simultaneously blurted out, "Which tire?" Urban legends
and persistent rumors are ubiquitous. Here are a few:
- The secret ingredient in Dr. Pepper is prune juice.
- A woman accidentally killed her poodle by drying it in a
microwave oven.
- Paul McCartney died and was replaced by a look-alike.
- Giant alligators live in the sewers of New York City.
- The moon landing was faked and filmed in a Hollywood
studio.
- George Washington had wooden teeth.
- The number of stars inside the "P" on Playboy magazine's cover indicates
how many times publisher Hugh Hefner had sex with the centerfold.
- A flying saucer crashed in New Mexico and the bodies of
the extraterrestrials are being kept by the Air Force in a secret
warehouse.
How many have you heard...and believed? None have ever been
confirmed.
10.
Unexplained Is Not Inexplicable
Many people are overconfident enough to think that if they cannot explain something, it
must be inexplicable and therefore a true mystery of the paranormal. An
amateur archeologist declares that because he cannot figure out how the
pyramids were built, they must have been constructed by space aliens.
Even those who are more reasonable at least think that if the experts cannot explain something,
it must be inexplicable. Feats such as the bending of spoons,
firewalking, or mental telepathy are often thought to be of a
paranormal or mystical natures because most people cannot explain them.
When they are explained, most people respond, "Yes, of course" or
"That's obvious once you see it." Firewalking is a case in point.
People speculate endlessly about supernatural powers over pain and
heat, or mysterious brain chemicals that block pain and prevent
burning. The simple explanation is that the capacity of light and
fluffy coals to contain heat is very low, and the conductivity of heat
from the light and fluffy coals to your feet is very poor. As long as
you don't stand around on the coals, you will not get burned. (Think of
a cake in a 450° oven. The air, the cake, and the pan are all at
450°F, but only the metal pan will burn your hand. Air has a very
low heat capacity and also low conductivity, so you can put your hand
in the oven long enough to touch the cake and pan. The heat capacity of
the cake is a lot higher than air, but since it has low conductivity
you can briefly touch it without getting burned. The metal pan has a
heat capacity similar to the cake, but high conductivity too. If you
touch it, you will get burned.) This is why magicians do not tell their
secrets. Most of their tricks are, in principle, relatively simple
(although many are extremely difficult to execute) and knowing the
secret takes the magic out of the trick.
There are many genuine unsolved mysteries in the
universe and it is okay to say, "We do not yet know but someday perhaps
we will." The problem is that most of us find it more comforting to
have certainty, even if it is premature, than to live with unsolved or
unexplained mysteries.
11. Failures Are Rationalized
In science, the value of negative finding findings - failures -
cannot be overemphasized. Usually they are not wanted, and often they
are not published. But most of the time failures are how we get closer
to the truth. Honest scientists will readily admit their errors, but
all scientists are kept in a like by the fact that their fellow
scientists will publicize any attempt to fudge. Not pseudoscientists.
They ignore or rationalize failures, especially when exposed. If they
are actually caught cheating - not a frequent occurrence - they claim
that their powers usually work but not always, so when pressed to
perform on television or in a laboratory, they sometimes resort to
cheating. If they simply fail to perform, they have ready any number of
creative explanations: too many controls in an experiment cause
negative results; the powers do not work in the presence of skeptics;
the powers do not work in the presence of electrical equipment; the
powers come and go, and this is one of those times they went. Finally,
they claim that if skeptics cannot explain everything, then there must
be something paranormal; they fall back on the unexplained
is not inexplicable fallacy.
12. After-the-Fact Reasoning
Also known as "post hoc, ergo
propter hoc," literally, "after this, therefore because of
this." At its basest level, it is a form of superstition. The baseball
player does not shave and hits two home runs. The gambler wears his
lucky shoes because he has won wearing them in the past. More subtly,
scientific studies can fall prey to this fallacy. In 1993 a study found
that breast-fed children have higher IQ scores. There was much clamor
over what ingredient in mother's milk increased intelligence. Mothers
who bottle-fed their babies were made to feel guilty. But soon
researchers began to wonder whether breast-fed babies are attended to
differently. Maybe nursing mothers spend more time with their babies
and motherly vigilance was the cause behind the differences in IQ. As Hume taught us, the fact that two events
follow each other in sequence does not mean they are connected
causally. Correlation does not mean causation.
13. Coincidence
In the paranormal world, coincidences are often seen as
deeply significant. "Synchronicity" is invoked, as if some mysterious
force were at work behind the scenes. But I see synchronicity as
nothing more than a type of contingency - a conjuncture of two or more
events without apparent design. When the connection is made in a manner
that seems impossible according to our intuition of the laws of
probability, we have a tendency to think something mysterious is at
work.
But most people have a very poor understanding of
the laws of probability. A gambler will win six in a row and then think
he is either "on a hot streak" or "due to lose." Two people in a room
of thirty people discover that they have the same birthday and conclude
that something mysterious is at work. You go to the phone to call your
friend Bob. The phone rings and it is Bob. you think, "Wow, what are
the chances? This could not have been a mere coincidence. Maybe Bob and
I are communicating telepathically." In fact, such coincidences are not
coincidences under the rules of probability. The gambler has predicted
both possible outcomes, a fairly safe bet! The probability that two
people in a room of thirty people will have the same birthday is .71.
And you have forgotten how many times Bob did not call under such
circumstances, or someone else called, or Bob called but you were not
thinking of him, and so on. As the behavioral psychologist B. F.
Skinner proved in the laboratory, the human mind seeks relationships
between events and often finds them even when they are not present.
Slot-machines are based on Skinnerian principles of intermittent
reinforcement. The dumb human, like the dumb rat, only needs an
occasional payoff to keep pulling the handle. The mind will do the rest.
14. Representativeness
As Aristotle said, The sum of the coincidences equals
certainty." We forget most of the insignificant coincidences and
remember the meaningful ones. Our tendency to remember hits and ignore
misses is the bread and butter of the psychics, prophets, and
soothsayers who make hundreds of predictions each January 1. First they
increase the probability of a hit by predicting mostly generalized sure
bets like "There will be a major earthquake in southern California" or
"I see trouble for the Royal Family." Then, next January, they publish
their hits and ignore the misses, and hope no on e bothers to keep
track.
We must always remember the larger context in which
a seemingly unusual event occurs, and we must always analyze unusual
events for their representativeness of their class of phenomena. In the
case of the "Bermuda Triangle," an area of the Atlantic Ocean where
ships and planes "mysteriously" disappear, there is the assumption that
something strange or alien is as work. But we must consider how
representative such events are in that area. Far more shipping lanes
run through the Bermuda Triangle than its surrounding area. As it turns
out, the accident rate is actually lower
in the Bermuda Triangle than in surrounding areas. Perhaps this area
should be called the "Non-Bermuda Triangle." (See Kusche 1975 for a
full explanation of this solved mystery.) Similarly, in investigating
haunted houses, we must have a baseline measurement of noises, creaks,
and other events before we can sat that an occurrence is unusual (and
therefore mysterious). I used to hear rapping sounds in the walls of my
house. Ghosts? Nope. Bad plumbing. I occasionally hear scratching
sounds in my basement. Poltergeists? Nope. Rats. One would be
well-advised to first thoroughly understand the probable worldly
explanation before turning to other-worldly ones.
Logical Problems in Thinking
15.
Emotive Words and False Analogies
Emotive words are used to provoke emotion and sometimes
to obscure rationality. They can be positive emotive words - motherhood, America, integrity, honesty.
or they can be negative - rape,
cancer, evil, communist. Likewise, metaphors and analogies can
cloud thinking with emotion or steer us onto a side path. A pundit
talks about inflation as "the cancer of society" or industry "raping
the environment." In his 1992 Democratic nomination speech, Al Gore
constructed an elaborate analogy between the story of his sick son and
America as a sick country. Just as his son, hovering on the brink of
death, was nursed back to health by his father and family, America,
hovering on the brink of death after twelve years of Reagan and Bush,
was to be nurtured back to health under the new administration. Like
anecdotes, analogies and metaphors do not constitute proof. They are
merely tools of rhetoric.
16. Ad
Ignorantiam
This is an appeal to ignorance or lack of knowledge and
is related to the burden
of proof and unexplained
is not inexplicable fallacies, where someone argues that if
you cannot disprove a claim it must be true. For example, if you cannot
prove that there isn't any psychic power, then there must be. The
absurdity of this argument comes into focus if one argues that if you
cannot prove that Santa Claus does not exist, then he must exist. You
can argue the opposite in a similar manner. If you cannot prove Santa
Claus exists, then he must not exist. In science, belief should come
from positive evidence in support of a claim, not lack of evidence for
or against a claim.
17. Ad Hominem and Tu Quoque
Literally "to the man" and "you also," these fallacies
redirect the focus from thinking about the idea to thinking about the
person holding the idea. The goal of an ad hominem attack is to discredit
the claimant in hopes that it will discredit the claim. Calling someone
an atheist, a communist, a child abuser, or a neo-Nazi does not in any
way disprove that person's statement. it might be helpful to know
whether someone is of a particular religion or holds a particular
ideology, in case this has in some way biased the research, but
refuting claims bust be done directly, not indirectly. If Holocaust
deniers, for example, are neo-Nazis or anti-Semites, this would
certainly guide their choice of which historical events to emphasize or
ignore. But if they are making the claim, for example, that Hitler did
not have a master plan for the extermination of European Jewry, the
response "Oh, he is saying that because he is a neo-Nazi" does not
refute the argument. Whether Hitler had a master plan or not is a
question that can be settled historically. Similarly for tu quoque. If someone accuses you
of cheating on your taxes, the answer "Well, so do you" is no proof one
way or the other.
18. Hasty Generalization
In logic, the hasty generalization is a form of improper
induction. in life, it is called prejudice. In either case, conclusions
are drawn before the facts warrant it. Perhaps because our brains
evolved to be constantly on the lookout for connections between events
and causes, this fallacy is one of the most common of all. A couple of
bad teachers mean a bad school. A few bad cars mean that brand of
automobile is unreliable. A handful of members of a group are used to
judge the entire group. In science, we must carefully gather as much
information as possible before announcing our conclusions.
19. Overreliance on Authorities
We tend to rely heavily on authorities in our culture,
especially if the authority is considered to be highly intelligent. The
IQ score has acquired nearly mystical proportions in the last half
century, but I have noticed that belief in the paranormal is not
uncommon among Mensa members (the high-IQ club for those top 2 percent
of the population); some even argue that their "Psi-Q" is also
superior. Magician James Randi is fond of lampooning authorities with
Ph.D.s - once they are granted the degree, he says, they find it almost
impossible to say two things: "I don't know" and "I was wrong."
Authorities, by virtue of the expertise in a field, may have a better
chance of being right in that field, but correctness is certainly not
guaranteed, and their expertise does not necessarily qualify them to
draw conclusions in other areas.
In other words, who
is making the claim makes a difference. If it is a Nobel laureate, we
take note because he or she has been right in a big way before. If it
is a discredited scam artist, we give a loud guffaw because he or she
has been wrong in a big way before. While expertise is useful for
separating the wheat from the chaff, it is dangerous in that we might
either (1) accept a wrong idea just because it was supported by someone
we respect (false positive) or (2) reject a right idea just because it
was supported by someone we disrespect (false negative). How do you
avoid such errors? Examine the evidence.
20. Either-Or
Also known as the fallacy
of negation or the false
dilemma, this is the tendency to dichotomize the world so that
if you discredit one position, the observer is forced to accept the
other. This is a favorite tactic of creationists, who claim that life either was divinely created or it evolved. Then they spend the
majority of their time discrediting the theory of evolution so that
they can argue that since evolution is wrong, creationism must be
right. But it is not enough to point out the weaknesses in a theory. If
your theory is indeed superior, it must explain both the "normal" data
explained by the old theory and the "anomalous" data not explained by
the old theory. A new theory needs evidence in favor of it, not just
against the opposition.
21. Circular Reasoning
Also known as the fallacy
of redundancy, begging the question, or tautology, this occurs when the
conclusion or claim is merely a restatement of one of the premises.
Christian apologetics is filled with tautologies: Is there a God? Yes. How do you know?
Because the Bible says so. How do you know the Bible is correct?
Because it was inspired by God. In other words, God is because
God is. Science also has its share of redundancies: What is gravity? The tendency for objects
to be attracted to one another. Why are objects attracted to one
another? Gravity. In other words, gravity is because gravity is.
(In fact, some of Newton's contemporaries rejected his theory of
gravity as being an unscientific throwback to medieval occult
thinking.) Obviously, a tautological operational definition can still
be useful. Yet, difficult as it is, we must try to construct operation
definitions that can be tested, falsified, and refuted.
22. Reductio
ad Absurdum and the Slippery Slope
Reductio ad absurdum
is the refutation of an argument by carrying the argument to its
logical end and so reducing it to an absurd conclusion. Surely, if an
argument's consequences are absurd, it must be false. This is not
necessarily so, though sometimes pushing an argument to its limit is a
useful exercise in critical thinking; often this is a way to discover
whether a claim has validity, especially if an experiment testing the
actual reduction can be run. Similarly, the slippery slope fallacy
involves constructing a scenario in which one thing leads ultimately to
an end so extreme that the first step should never be taken. For
example: Eating Ben & Jerry's
ice cream will cause you to put on weight. Putting on weight will make
you overweight. Soon you will weigh 350 pounds and die of heart
disease. Eating Ben & Jerry's ice cream leads to Death. Don't even
try it. Certainly eating a scoop of Ben & Jerry's ice cream may contribute to obesity, which
could possibly, in very rare cases, cause death. but the consequence
does not necessarily follow from the premise.
Psychological Problems in
Thinking
23.
Effort Inadequacies and the Need for
Certainty, Control, and Simplicity
Most of us, most of the time, want certainty, want to
control our environment, and want nice, neat, simple explanations. All
this may have some evolutionary basis, but in a multifarious society
with complex problems, these characteristics can radically oversimplify
reality and interfere with critical thinking and problem solving. For
example, I believe that paranormal beliefs and pseudoscientific claims
flourish in market economies in part because of the uncertainty of the
marketplace. According to James Randi, after communism collapsed in
Russia there was significant increase in such beliefs. Not only are the
people now freer to try to swindle each other with scams and rackets
but many truly believe they have discovered something concrete and
significant about the nature of the world. Capitalism is a lot less
stable a social structure than communism. Such uncertainties lead them
mind to look for explanations for the vagaries and contingencies of the
market (and life in general), and the mind often takes a turn toward
the supernatural and paranormal.
Scientific and critical thinking does not come
naturally. It takes training, experience, and effort, as Alfred Mander
explained in his Logic for the
Millions: "Thinking is skilled work. It is not true that we are
naturally endowed with the ability to think clearly and logically -
without learning how, or without practicing. People with untrained
minds should no more expect to think clearly and logically than people
who have never learned and never practiced can expect to find
themselves good carpenters, golfers, bridge players, or pianists"
(1947, p.vii). We must always work to suppress our need to be
absolutely certain and in total control and our tendency to seek the
simple and effortless solution to a problem. Now and then the solutions
may be simple, but usually they are not.
24. Problem-Solving Inadequacies
All critical and scientific thinking is, in a fashio,
problem solving. There are numerous psychological disruptions that
cause inadequacies in problem solving. Psychologist Barry Singer has
demonstrated that whn people are given the task
of selecting the right answer to a problem after being told whether
particular guesses are right or wrong, they:
A. Immediately form a hypothesis and look only for examples to confirm
it.
B. Do not seek evidence to disprove the hypothesis.
C. Are very slow to change the hypothesis even when it is obviously
wrong.
D. If the information is too complex, adopt overly-simple hypothesis or
strategies fro solutions.
E. If there is no solution, if the probllem is a trick and "right" and
"wrong" is given at random, form hypothesis about coincidental
relationships they observed. Causality is always found. (Singer and
Abell 1981, p.18)
If this is the case with humans in gneral, then we all
must make the effort to overcome these inadequacies in solving the
problems of science and of life.
25. Ideological Immunity, or the Planck
Problem
In day-to-day life, as in science, we all resist
fundamental paradigm change. Social scientist Jay Stuart Snelson calls
this resistance an ideological
immune system: "educated, intelligent, and successful adults
rarely change their most fundamental presuppositions" (1993, p.54).
According to Snelson, the more knowledge individuals have accumulated,
and the more well-founded their theories have become (and remember, we
all tend to look for and remember confirmatory evidence, not
counterevidence), the greater the confidence in their ideologies. The
consequence of this, however, is that we build up and "immunity"
against new ideas that do not corroborate previous ones. Historians of
science call this the Planck Problem,
after physicist Mac Planck, who made this observation on what must
happen for innovation to occur in science: "An important scientific
innovation rarely makes its way by gradually winning over and
converting its opponents: it rarely happens that Saul becomes Paul.
What does happen is that its opponents gradually die out and that the
growing generation is familiarized with the idea from the beginning"
(1936, p.97).
Psychologist David Perkins conducted an interesting
correlational study in which he found a strong positive correlation
between intelligence (measured by a standard IQ test) and the ability
to give reasons for taking a point of view and defending that position;
he also found a strong negative correlation between intelligence and
the ability to consider other alternatives. That is, the higher the IQ,
the greater the potential for ideological immunity. Ideological
immunity is built into the scientific enterprise, where it functions as
a filter against potentially overwhelming novelty. As historian of
science I. B. Cohen explained, "New and revolutionary systems of
science tend to be resisted rather than welcomed with open arms,
because every successful scientist has a vested intellectual, social,
and even financial interest in maintaining the status quo. If every
revolutionary new idea were welcomed with open arms, utter chaos would
be the result" (1985, p.35).
In the end, history rewards those who are "right"
(at least provisionally). Change does occur. In astronomy, the
Ptolemaic geocentric universe was slowly displaced by Copernicus's
heliocentric system. In geology, George Cuvier's catastrophism was
gradually wedged out by the more soundly supported uniformitarianism of
James Hutton and Charles Lyell. In biology, Darwin's evolution theory
superseded creationist belief in the immutability of species. In Earth
history, Alfred Wegener's idea of continental drift took nearly a half
century to overcome the received dogma of fixed and stable continents.
Ideological immunity can be overcome in science and in daily life, but
it takes time and corroboration.
Spinoza's Dictum
Skeptics have the very human tendency to relish debunking what
we already believe to be nonsense. It is fun to recognize other
people's fallacious reasoning, but that's not the whole point. As
skeptics and critical thinkers, we must move beyond our emotional
responses because by understanding how others have gone wrong and how
science is subject to social control and cultural influences, we can
improve our understanding of how the world works. It is for this reason
that it is so important for us to understand the history of both
science and pseudoscience. If we see the larger picture of how these
movements evolve and figure out how their thinking went wrong, we won't
make the same mistakes. The seventeenth-century Dutch philosopher
Baruch Spinoza said it best: "I have made a ceaseless effort not to
ridicule, not to bewail, not to scorn human actions, but to understand
them."
Section VII - Stuff on the Web